Research Theme within School of Biosciences: Microbiology and Infection
Lab website address: www.biosciences-labs.bham.ac.uk/kreftlab/index.html
Cooperation and communication of microbes in biofilms, metabolic division of labour, individual-based modelling, systems biology
Dynamics of interaction
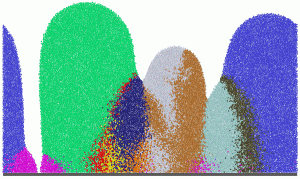
I'm broadly interested in the dynamics of interaction between 'parts' (e.g. individual organisms) and how these interactions give rise to emergent behaviour on the next higher level of organisation (e.g. the population). There are far more fascinating examples than one can possibly study, so I have focused on competition, cooperation, and communication of microbes in spatially structured systems such as biofilms.
How the simple process of substrate diffusion and biomass growth leads to complex biofilm structures can be seen in the image of a nitrifying biofilm above, which shows different clones of ammonia oxidizers in different colours and all clones of nitrite oxidizers in magenta. The competition for oxygen diffusing in from above leads to the formation of finger-like structures and lateral inhibition (in this case suffocation). This biofilm structure was generated by an Individual-based Model that describes the behaviour and activities of the individual microbes and how they interact with each other and their environment. This model treats each individual bacterial cell as a hard ball, following the spirit of considering a spherical cow. See the individual-based modelling of biofilms paper.
Below are some examples of my current research projects, for more details and other projects please visit the Kreft lab pages.
Evolution of cooperation in biofilms
We are studying a case of cooperation that consists in the economic use of resources, known as the 'tragedy of the commons' and have shown that this cooperation can evolve in biofilms. The benefit of saving common and limiting resources is indiscriminately but locally shared among the neighbourhood while the more efficient metabolism comes at the cost of a reduced specific growth rate of the cooperating individuals due to a thermodynamic trade-off between biomass yield per amount of resource and growth rate.

Using an individual-based model, the competition between wasteful (blue) and efficient (red) individual bacteria can be simulated in spatially complex systems such as biofilms.
It turns out that spatial structure is of key importance for the success of the slower but more efficient bacteria. The importance of spatial structure can be best appreciated watching movies of model simulations, for further explanations please see the altruism paper.
Twitching motility and biofilm structure
Some bacteria like Pseudomonas aeruginosa can pull themselves across surfaces by the use of type IV pili as grappling hooks (more on Alex Merz's web site). This twitching motility called process has remarkable effects on biofilm structure in P. aeruginosa because a wild type population phenotypically separates into an immotile and motile subpopulation. 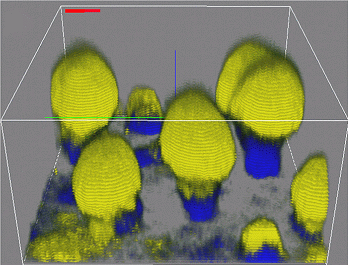
The immotile subpopulation forms clonal microcolony stalks and the motile subpopulation appears to crawl up on the stalks to form caps, allowing the population as a whole to form the mushroom structures you can see in the micrograph (the image is from a biofilm including wild type in yellow and an immotile mutant in blue for a more reliable separation of the subpopulations).
This phenotypic split has some interesting evolutionary consequences we have briefly discussed in a recent review.
We have been developing an individual-based model of biofilms including twitching motility to dissect the processes involved and to understand the fitness effects of this behaviour, for a description see our paper.
This project has been a collaboration with Cristian Picioreanu, at the Delft University of Technology, The Netherlands, and Mikkel Klausen, Janus Haagensen, Tim Tolker-Nielsen, Søren Molin, at the Danish Technical University in Lyngby near Copenhagen.
Horizontal gene transfer (HGT) in biofilms
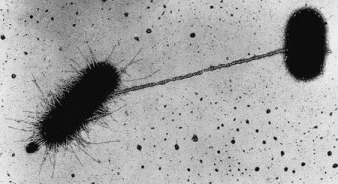
The horizontal transfer of genes from individual to individual by conjugation (see electron microscopic image by Charles C. Brinton, Jr., of a mating pair initially brought together by means of an F pilus), or via extracellular DNA by transformation, is a remarkable and prevalent phenomenon in bacterial communities, where the spatial arrangement of donor and recipient is obviously important.
This calls for the use of spatially explicit models that allow the status and activities of the individuals to change due to gene transfer, in other words, individual-based models. We are developing such an individual-based model of conjugation that we will use to predict HGT in biofilms and also to investigate the evolutionary and ecological consequences of HGT.
This project is a collaboration with Barth F Smets' group at the Danish Technical University in Lyngby near Copenhagen. Our ‘next-generation’ individual-base model we have been developing for this and many future projects is called individual-based simulator for Dynamics of Microbial Communities Simulator (iDynoMiCS). It was originally developed by Laurent Lardon and then Brian Merkey, with contributions to the design and/or programming by Sonia MArtins, Cristian Picioreanu, Andreas Dötsch, Joao Xavier, Barth F Smets, and myself.
One-step nitrification
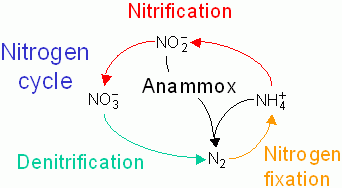
Since Winogradsky's discovery in 1890, it is known that nitrification, an important link in the global Nitrogen cycle, is carried out by two physiologically and phylogenetically distinct groups of bacteria, the first step is the incomplete oxidation of ammonia (NH4+) to nitrite (NO2-), which is oxidized further to nitrate (NO3-) by a second group of bacteria. Stahl and coworkers recently discovered that the first step can also be carried out by archaea.
Why doesn't the first group of bacteria or archaea oxidize ammonia completely? We have argued that this metabolic division of labour can be explained by kinetic theory of optimal pathway length, which assumes that the production of enzymes as well as the presence of intermediates is costly and that the number of ATP generating steps is proportional to the length of the pathway. From these assumptions, the existence of an optimal pathway length follows which maximises the rate of ATP production. Shortening the pathway by oxidizing ammonia only incompletely could therefore increase the rate of ATP production and growth, increasing fitness in environments such as enrichment cultures that select for fastest growth rate.
However, there is a trade-off between growth rate and growth yield, since the longer pathway would have more ATP generating steps, thereby increasing growth yield, which we have shown (see the cooperation section above) to be advantageous when bacteria grow in clonal clusters as is typical for biofilms. We therefore postulate the existence of bacteria that completely oxidize ammonia to nitrate and have suggested to use biofilm cultures to select for the higher yield that these "lithotrophs missing in nature" (Broda, 1977) should have. We are interested in isolating and constructing such bacteria by genetic engineering and in silico.
See our opinion paper for an explanation of kinetic theory and further examples of microbial food webs with versus without division of labour. A further paper has analyzed the conditions under which a food-chain carrying out complete ammonia oxidation is advantageous compared to incomplete ammonia oxidation (see partial nitrification paper).
This project has been a collaboration with Julio Pérez, Dpt. d'Enginyeria Química, Universitat Autònoma de Barcelona, Spain.
Cell-cell communication
Bacteria are simple organisms without brains, but they nevertheless evolved some fascinating social behaviours, for example cell-cell communication by production of diffusible signal molecules called autoinducers. This has been studied a lot in well-mixed liquid cultures, where the concentration of autoinducers depends on the density of cells, and this has led to the idea that cells indeed use these autoinducers for the control of gene expression by the density of cells in a population, known as quorum sensing. However, in most natural habitats, the complex physical structure of the environment and the clustered distribution of cells growing on surfaces (think soil), can have a stronger effect on the concentration of autoinducers a given cell experiences than the density of cells.
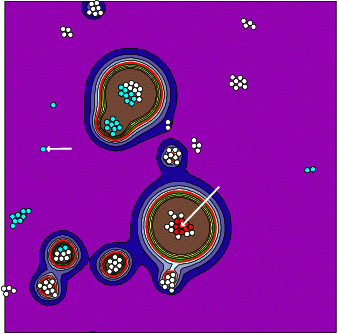
The figure shows results of a simulation, using iDynoMiCS, by my former student Andreas Dötsch. The distribution of cells (circles) is clustered and the contours show the autoinducer concentration (colour scheme as in a topographic map: blue is below threshold (below sea level), red contour line = threshold concentration, green and brown = islands of upregulation, the shore is green and mountains are brown). You can see that small clusters of autoinducer producing cells easily become upregulated while lone cells don't. Further, the autoinducer that the focal red cell in the microcolony experiences has been produced by itself and its close neighbours only (all the red cells), while the focal lone cell in cyan listens mostly to itself and a variety of other cells, all in cyan.
This shows that communication can be kept private to microcolonies, and that has important evolutionary consequences because microcolonies are clones, all cells are offspring of a single founding cell.
See our opinion paper for a critical analysis of quorum sensing problems in the natural habitat and our proposed solution.
This project has been a collaboration with my colleagues Burkhard A. Hense, Christina Kuttler, and Johannes Müller from the Institute for Biomathematics and Biometry (IBB) and Michael Rothballer and Anton Hartmann from the Department Microbe-Plant-Interaction, both based at the GSF - National Research Center for Environment and Health, near München, Germany.