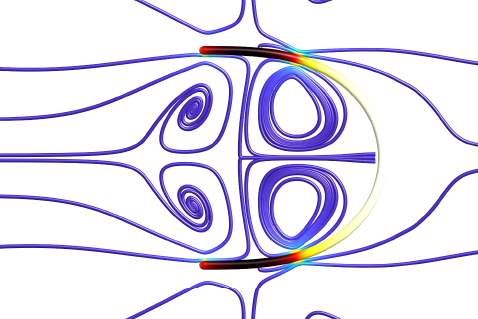
The past 15 years have seen an explosion in research into the creation of microscale robots to perform a variety of jobs. Developments in materials and manufacturing technologies have worked hand in hand with theoretical advances in understanding microscale propulsion.
But when it comes to micromachines, or microbots, that can swim – necessary for precision tasks such as targeted drug delivery – there is a major stumbling block: microscale swimming is a very different process to human-scale swimming and, as a result, effectively controlling individual microbots within a large group, or swarm, has so far proved impossible.
To overcome this obstacle, mathematical biologist Dr Tom Montenegro-Johnson has turned to nature – and hit on the idea of replicating the way bacteria swim and navigate. As they propel themselves through their environment, certain bacteria such as E.coli move in a series of straight runs followed by on-the-spot reorientations.
For the first time, he has been able to show that it should be possible to mimic this behaviour by making microbots that selectively transform between different shapes to control a group of microbots to do different things.
Illustration: schematic demonstrating the need for independent control of robots at the T-Junction of a microfluidic device and how that would be achieved with targeted shape change of microtransformers

His findings are explained in a paper entitled ‘Microtransformers: Controlled microscale navigation with flexible robots’, which was published in the journal Physical Review Fluids and recently won Tom the College’s Paper of the Month award. It’s the first paper to come out of a two-year, EPSRC-funded project whose next goal is to develop a lab prototype.
‘The strategies you need to swim if you’re human are very different to those you need if you’re an E.coli bacterium,’ explains Tom, a Lecturer in Applied Mathematics. ‘If an E.coli bacterium stops beating its tail, it stops instantly, because the major force at play is viscosity. If a human stops swimming, they carry on gliding for a bit, because for us the force of inertia is more powerful.
‘Since scientists started making artificial microswimmers, or microbots, there have been all sorts of advances and we’re now at the stage where we can categorise them into either externally driven – usually driven by a magnetic field – or fuel-driven, where fuel is taken from their surroundings in order to self-generate propulsion.’
There are problems with both, however: Externally driven microswimmers swim along user-defined paths, so if you want them to turn left, you alter the magnetic field accordingly. The disadvantage is that if you have multiple swimmers, they will all turn left; it’s not possible to control individuals within a group. The downside of fuel-based microbots is that there’s no user control – their trajectories arise essentially from their physical interactions with their boundaries and other microbots.
‘So there remains this fundamental problem of controlling individuals in a swarm,’ says Tom.
Various control strategies have been proposed, such as putting small grooves in microchannels to guide fuel-driven swimmers, ‘but the problem with that is you guide all of them the same way. Also, you can’t start etching grooves in people’s bodies!’
So, Tom came at the problem from a different angle. Instead of changing the physical environment to guide the fuel-based swimmer, why not change the fuel-based swimmer’s shape in order to modify its swimming behaviour?
Using new mathematical tools, Tom showed it was possible to mimic bacterial ‘run-and-tumble’ navigation to make ‘microtransformers’ or ‘transforming microbots’ that could be controlled effectively even when within a group – thus paving the way for radical biomedical applications such as delivering chemotherapy directly to the site of tumours and heart patients a simple injection instead of surgery.
‘You take a soft, flexible filament made of thermo-responsive material with both ends coated in platinum, and you design it in such a way that heating it up transforms it into a shape that would give you different swimming behaviour,’ he explains. ‘When placed in hydrogen peroxide, the platinum will catalyse its reduction into water and oxygen, causing a flow at the surface of the filament. If the filament is straight, this flow would act as a pump; if bent in a "U" shape, the filament would translate – or move a certain distance – and if bent into an "S" shape, it would rotate on the spot.
‘So, by selectively heating up these swimmers, using a laser, you can get them to navigate to where you want them to go, then cool them down so they change shape again and go off in a different direction.’
Tom concedes there is still a long way to go before swimming micromachines are biocompatible enough to use in healthcare and microfluidics applications – but he’s confident it will happen.
‘Developing a prototype of these microtransformers is a complex task that spans traditional scientific disciplines – how do you heat up the microbots safely in the human body, for example? – and it will only be possible with new mathematical theories and cutting-edge materials that can be "programmed" to remember specific shapes,’ he observes. ‘But by the end of the project, this prototype will be sufficiently developed to begin commercialisation of the technology for industrial use, and to begin the development of a biocompatible prototype for tiny robots that go into your body and heal you from the inside out.’