by Nigel Cassidy, Ian Jefferson, David Chapman, Nicole Metje, Chris Rogers, Jon Roberts and Paul O'Regan
A 2020 report by the UN Environment Programme (UNEP) estimated that the building sector accounts for a staggering 38% of world-wide energy-related CO2 emissions and that the construction industry is responsible for nearly 30% of this figure. The report also highlights the increasing levels of emissions from the sector with 2019 reaching an all-time high of almost 10 GigaTonnes of equivalent CO2. As such, the construction industry is, quite rightly, under pressure from the UK government to change practices and meet net zero carbon targets by 2050.
Organisations such as the UK Green Building Council are at the forefront of initiatives to reduce energy use and embedded carbon cost across the whole building sector. Understandably, the focus is on reducing emissions from the buildings themselves, be it during construction or over their whole lifecycle, and the embedded carbon cost of the construction materials is a very important part of this carbon footprint calculation. Whole-life embedded carbon assessment is nothing new and the Institution of Civil Engineers (ICE) originally produced embedded carbon data and calculation guides back in 2011. Most major public building projects are now required to calculate the “cradle to gate” and/or “cradle to grave” carbon impact as part of the design process. This is all good news, and the UK leads the world in the integrity and depth of its embedded carbon assessment processes. Nevertheless, are we doing enough to reduce carbon impact across the sector and are there missing elements to the analysis – a hidden cost, say, that is absent from the assessment? We believe there is something missing.
The embedded carbon cost in equivalent kilograms of CO2 emissions per kilogram of material kgCO2e/kg) is traditionally calculated for specific individual materials (cast concrete, say) but in reality, there are a multitude of choices (e.g., different concrete grades). It requires complex calculation routes to come up with an overall figure for a construction project and it becomes a numbers game (which suits engineers) to reduce the carbon footprint against project cost. It is also worth noting that profit margins in the construction sector are 2% at best and, therefore, reducing the embedded carbon cost on site is not a priority to most contractors.
Ultimately, there has to be some standard process for embedded carbon evaluation and good quality evidential information is vital for accurate calculations. The material-focused assessment is the best approach we have at the moment, but it does not account for the whole carbon cost of the development as, normally, the infrastructure systems feeding the buildings (water, gas, electricity, drainage, etc.) are not included in the life-cycle carbon assessment. Take water supply and drainage infrastructure as an example. There are estimated to be over 600,000 kilometres of buried water, sewage and drainage pipes in the UK that, ultimately, need replacing over a cycle of about 50 years. This is easily in the “cradle to grave” lifetime of a building but the carbon cost of this supporting infrastructure is not included in the overall carbon assessment – we argue it should be.
Modern-day pipes, sewers and water drainage systems are predominantly made of plastic, usually high-density polyethylene (HDPE) or polyvinylchloride (PVC), ceramic or cast and/or reinforced concrete. These materials have a high embedded carbon cost (see Figure 1a) but considerable effort is being made by manufacturers to reduce the carbon cost of their products. Through the use of 100% recycled plastics and reduced carbon concrete mixtures these ‘low-carbon’ alternatives have claimed embedded carbon reductions of between 50 to 80%. Good news, but the numbers do not tell the full story. By focusing on the primary construction material (the pipe in this case), the embedded cost of installation, including the excavation of soil and backfilling, is an afterthought in the carbon reduction process - essentially out of sight and out of mind. Under current pipeline installation standards, fresh aggregate materials (usually sand and/or gravel) are required for the fill materials around the pipe in order to guarantee installation integrity and quality – it is a pipe-soil system that is being provided. As such, there is a significant added carbon cost in the excavation and off-site removal of the original soils and the import of fresh aggregates to backfill the pipe trench and reinstate the surface.
These ‘virgin’ aggregates have an embedded carbon cost attributed to them (see figure 1a again) which is predominantly related to the transport cost of delivery to site. However, this is at least a 100 times less than the embedded carbon cost of the pipe materials themselves (concrete, plastic, etc.) and, consequently, the impact of using virgin fill materials is commonly overlooked in the overall carbon budget. The real carbon cost needs to take into account the true volume of the pipe trench (and therefore the volume of embedment and backfill materials) and the carbon cost of removing the excavated ‘as dug’ materials from site. A better way to look at the assessment is to take a “whole installation” view and define the embedded carbon per unit length of pipe installation (per 100m, say). This puts a different perspective on the overall carbon cost (see Figure 1b) and for a typical, 300mm diameter drainage pipe installation, would mean that the carbon cost associated with virgin aggregate use is almost equivalent to that of the precast concrete pipe itself. If the estimated 50-80% carbon reduction figures stated for low-carbon plastic and concrete pipes can be realised, then the highest carbon cost for any new pipe installation is likely to be associated with the backfill/embedment materials and trench reinstatement – a fact that might surprise some contractors in the pipeline sector.
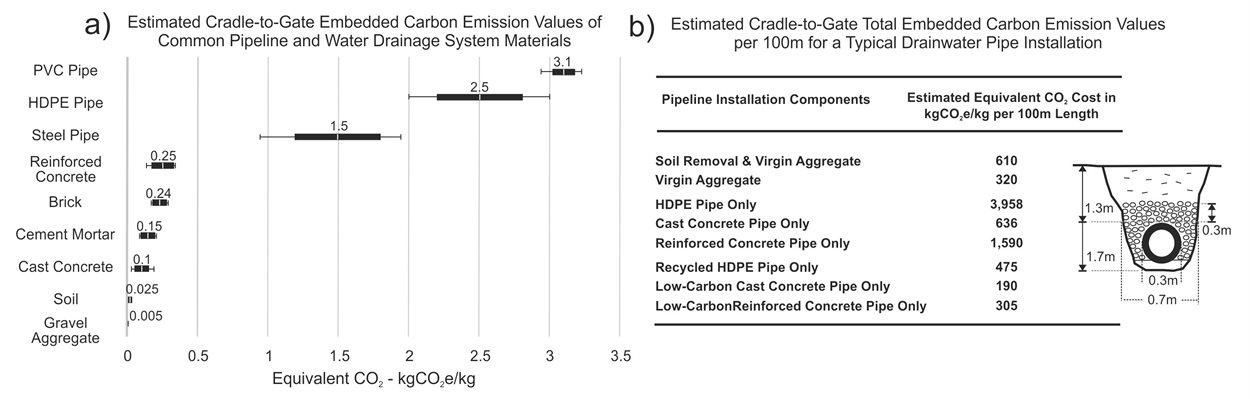
Figure 1. Estimated cradle-to-gate embedded carbon emission values for pipeline materials and typical pipeline installation components.
So, what can be done to meet our net carbon goals?
Obtaining alternative backfill/embedment materials from recycled sources is good start but using the excavated ‘as dug’ material from site is a better, more sustainable option. The materials will need careful sorting and perhaps processing on site, which will increase the monetary cost, but transport-related savings should balance this.
Research being undertaken under the University of Birmingham’s ARLI programme (Alternative Raw materials with Low Impact) is already investigating how the sector can better use its waste streams (e.g., using recycled concrete as the embedment material). More interestingly, waste and by-product streams from the non-construction industries are also being evaluated including crushed glass, manmade fibres and even eggshells. Incorporating these alternative resources into the ‘as-dug’ materials on site helps condition and modify the physio-chemical properties of the excavated soils, ensuring a more even and stable backfill for the pipeline.
Regulations are also a barrier with current standards for pipeline installation not readily accommodating recycled embedment materials in their scope. Regulation change takes a long time but a working group of the Pipeline Industries Guild has been set up to tackle the issue head on. Consisting of members from academic groups, including the University of Birmingham, industry research centres (e.g., Water Research Centre) and highly experienced consultants, the aim is to transform pipeline installation practices which will then inform changes to current standards (e.g., BS 9295:2020 Guide to the structural design of buried pipes) with carbon reduction as the focus. The aim is to cut out ‘off-site’ material transport and eliminate the need for bought-in embedment materials (particularly virgin aggregates). Summed up in Figure 2, our initial research indicates that a saving of ~70% in embedded carbon could be made with practices transformed by 2030. Not quite net zero, but a long way down the road to the 2050 target.
The pipeline manufacturers are playing their part as well. In a current InnovateUK funded research programme, Aquaspira Ltd are working with the University of Birmingham to develop almost 100% recycled composite steel and HDPE “SmartSense” pipes that can be installed with recycled or as-dug embedment materials and embedded sensing to autonomously monitor ground conditions. Key to this research is developing a better understanding of the detailed physical and geotechnical properties of the embedment material and how the whole pipeline installation performs when ground loading conditions change around the pipe.
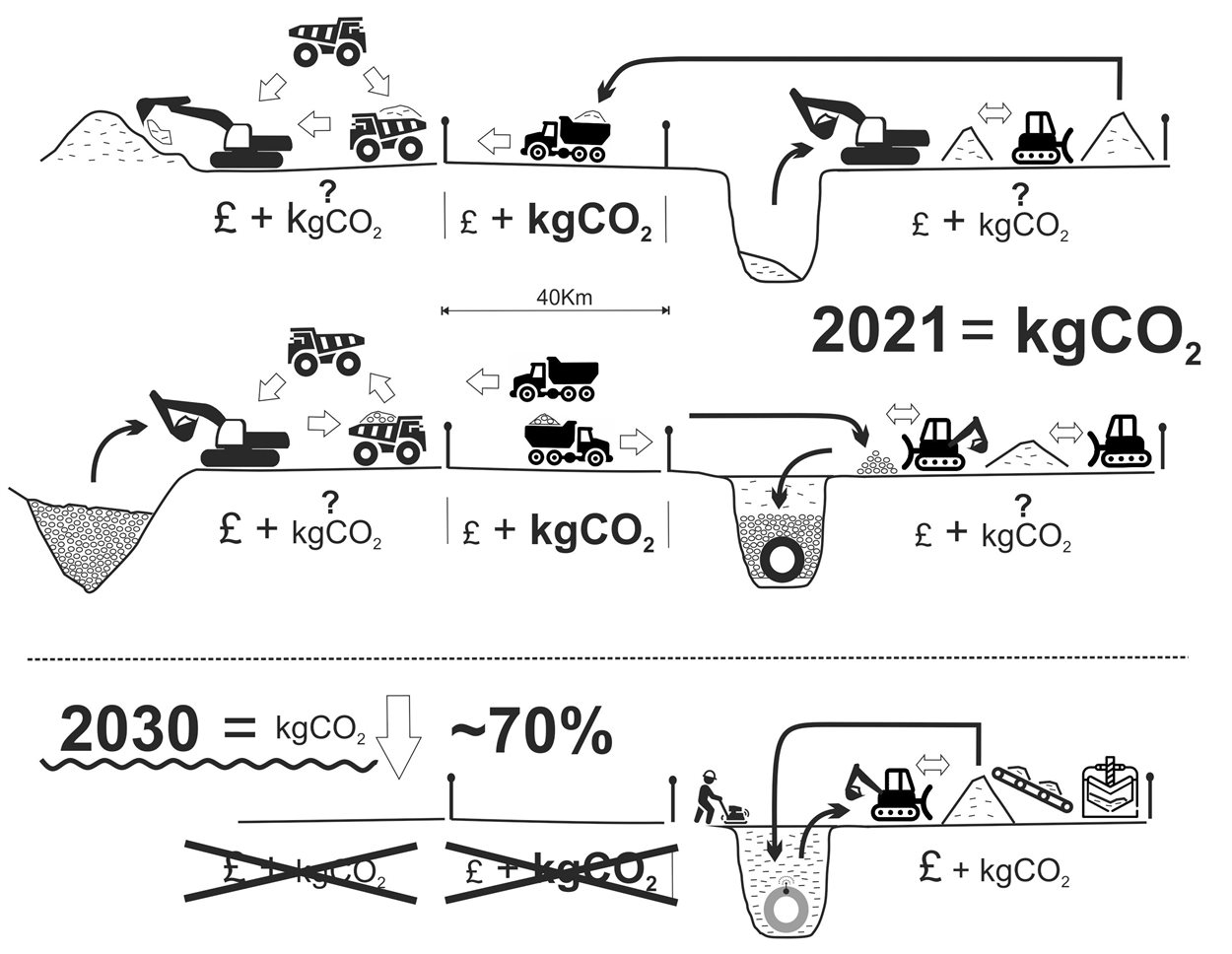
Figure 2. Reducing embedded carbon cost for pipeline installations – a move to using on-site materials and reduced transport.
Computational technology is helping in this regard with sophisticated numerical models being developed to create a digital simulant (or ‘digital twin’) of full-scale pipe installations. The research is utilising the testing capabilities of the new, £27millon UKCRIC National Buried Infrastructure Facility (NBIF) located on the University of Birmingham’s Edgbaston campus. Full-scale trials will be conducted with the SmartSense pipes where ground and performance data will be used to develop and optimise the performance of the digital twin co-product. The UK is world-leading in the development of national digital twin capability and digital simulators will play an important part in establishing the baseline data needed to reduce our carbon impact.
A recently released Royal Society policy briefing on “computing for net zero” recognises the contribution that Digital Twins can have towards meeting net zero goals; it states, “Digital twins make it possible to establish feedback loops to monitor, understand, optimise and reduce GHG emissions in many sectors”. The work Aquaspira and the University are undertaking is just part of this digital infrastructure revolution with BIM (Building Information modelling) already being used to evaluate entire building life-cycle performance and carbon budgets from inception and design through to demolition and materials reuse. The evolution of BIM into whole-site and even city-scale digital twins will transform our long-term management of energy, water and waste and provide the underpinning technology to meet the data needs for a net zero sector. It is a very exciting time to be part of the buried infrastructure engineering community and the goal of net zero by 2050 is a challenging one, but very much achievable.