Micromanipulation
There are many functional products containing biological and non-biological microscopic particles (or microparticles) over a wide range of industrial sectors including chemical, agrochemical, food and feed, pharmaceutical and medical, human care and household care. For biological microparticles, understanding their mechanical properties under different physiological states is crucial to bioprocessing and tissue engineering, e.g. animal cell culture to produce monoclonal antibodies, mechanical disruption of yeast and bacteria to extract intracellular proteins, and mechanical stimulation of chondrocytes for cartilage tissue engineering. Non-biological microparticles should have desirable chemical composition, structures and mechanical properties. Understanding the mechanical properties of such microparticles is essential to predicting their behaviour in manufacturing, handling and performance in end-use applications.
Over the last twenty-five years, novel micromanipulation techniques have been developed by the of Micromanipulation and Microencapsulation Group at the University of Birmingham, led by Professor Zhibing Zhang, to measure the mechanical properties of single microparticles, including animal cells, yeast, bacteria, pollen grains, microspheres and microcapsules, particle-particle adhesion, and to investigate particle adhesion on surfaces, adhesion and cohesion of biofilms or food fouling deposits on surfaces. The techniques have been applied to characterise the mechanical properties of these biological and non-biological materials, which are related to their structures and functions.
The basic principle of micromanipulation is the compression of a single particle (for example, a cell in a drop of medium) between two parallel surfaces. These are usually the flat end of a glass probe and the bottom surface of a sample chamber or a microscope slide surface. Figure 1 shows a single tomato cell being compressed. As the probe is driven by a micromanipulator towards the slide, a transducer measures the force being imposed on the particle. A force-displacement curve can be generated from the data. Unlike other methods, large deformations are possible, including to bursting for cells. Mathematical modeling of the force-displacement data based on analytical or finite element analysis can be applied to determine the intrinsic mechanical property parameters of the particle materials, e.g. Young’s modulus, Poisson ratio, yield stress and stress at failure.
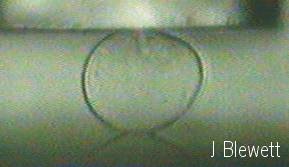
Figure 1: Single plant cell positioned between a force probe and glass slide. Cell size ca. 50 μm.
Microencapsulation
In addition, the Micromanipulation and Microencapsulation Group has established a research area in formulation of particulate functional products for pharmaceutical, nutraceutical, oral care and fabric care applications based on micro/bioencapsulation. For example, dextran-hydroxy-ethyl-methacrylate microspheres with a protein drug embedded, which may be used to achieve sustained release in blood stream, are shown in Figure 2. Different functional microparticles, with a matrix structure (microsphere, Figure 2) or core/shell structure (microcapsules), have been formulated using a range of techniques, including polymerization, coacervation, solvent evaporation, extrusion/gelation, fluidised bed coating, and direct compaction. The active ingredients which have been encapsulated included oil soluble droplets, water soluble powders, nutraceutical enzyme and probiotic cells.
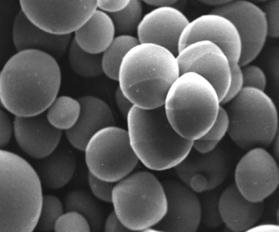
Figure 2: Dextran-hydroxy-ethyl-methacrylate microspheres prepared by emulsion polymerisation for sustained drug delivery.
Figure 3: Cellulose acetate butyrate microspheres loaded with cetyl pyridinium chloride crystals prepared by solid-in-oil-in-oil emulsion solvent evaporation emulsion with amorphous magnesium-calcium carbonate nanoparticles (Mg-CaCO3) as stabilizer.
Figure 4: TEM images showing wall thicknesses and the degree of wall completeness of ripened double-shell composite perfume microcapsules prepared using a method based on in situ polymerization of melamine Formaldehyde (MF) with a copolymer migrating though the interstice of the ripened CaCO3 shell, and polymerizing at the oil–water interface inside the ripened CaCO3 nanoparticulate shell.
Recent work includes development of a novel nanomanipulation technique to measure the mechanical properties of single nano-particles and to understand the rupture mode of microcapsules (Figure 3) in collaboration with Professor A. M. Donald, FRS, at Cavendish Laboratory, University of Cambridge, and the formulation of smart microcapsules for controlled release of small molecules in collaboration with Professor J. Preece, School of Chemistry, University of Birmingham.
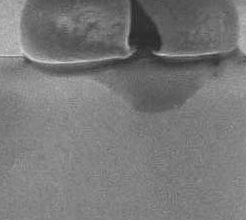
Figure 5: The image of a M-F microcapsule after it was compressed to rupture under high vacuum of ESEM (5kV, spot size 4). The diameter of the microcapsule was 16.50 µm.