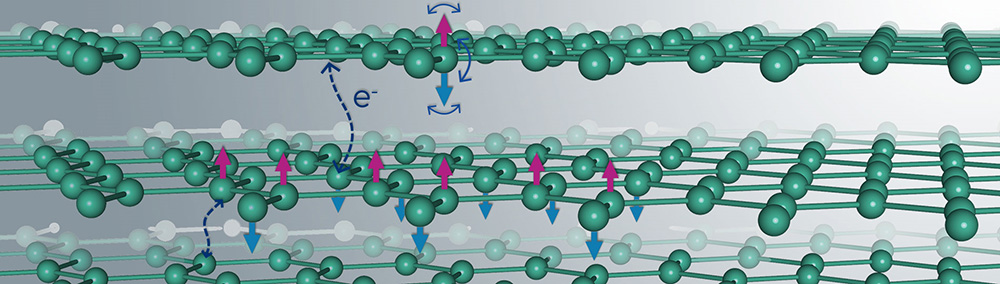
The Coak group specialises in extreme conditions tuning of two-dimensional materials. This means subjecting crystals of interesting new compounds to ultra-high pressures and magnetic fields, all at temperatures orders of magnitude below those of interstellar space. We search for new functional quantum materials and tune existing systems into novel states, focussing on fundamental properties of transport and of magnetic and charge order. After understanding the physics in bulk crystals, we then seek to fabricate and tune nanoscale devices built from stacking individual atomic layers of different 2D materials together.
PhD and postdoc opportunities
2D Materials are the future – graphene was just the beginning and the possibilities before us now are endless. We aim to understand the full range of properties in exciting new 2D materials - so you will be exposed to a wide toolbox of techniques: building and tweaking cryogenic equipment, programming computers, growing new crystals, measuring their properties in the lab & travelling to X-ray and neutron User Facilities around the world for experiments. You will get your hands dirty and gain expertise in low-temperature and ultra-high-pressure measurement techniques – both highly desirable skills for further research careers. Please email Dr Matt Coak at the address below to arrange a discussion. Open PhD studentships are listed on the central University Find a PhD page, but informal discussions are welcomed at any time.
Useful links
Dr Matt Coak's staff page
Dr Matt Coak's research portal
Contact: m.j.coak@bham.ac.uk
Research
High pressure measurements
We specialise in the use of diamond anvil cells and piston-cylinder cells to measure a wide variety of properties of materials under hydrostatic pressure.
Applying ultra-high hydrostatic pressure to materials allows a high degree of tunability of interactions and exploration of new phases of matter. This is particularly powerful in 2D materials, where inter-planar interactions are selectively strengthened via pressure as the material gradually becomes more 3D. We then have the opportunity to study not just 2D or 3D materials, but ‘2.5D’ – where there are more questions than answers. This regime also greatly favours the formation of new cooperative electronic states – it is no coincidence that high-temperature superconductivity is found in materials exhibiting a ‘2.5D’ nature.
We measure electrical transport, capacitance and magnetisation of single crystals down to low temperatures (~100 mK) and high magnetic fields in the lab. Beyond this, we also measure crystal and magnetic structures via X-ray and neutron scattering at external facilities, under these extreme conditions.
Quantum criticality
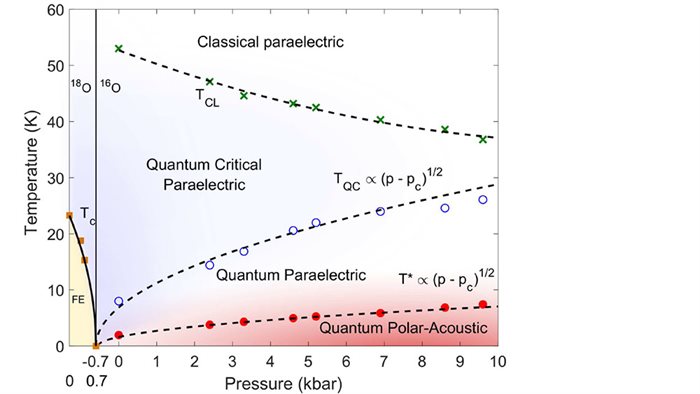
As well as dimensionality control, another powerful tool when seeking new phases of matter is searching in regimes dominated by quantum critical fluctuations. A quantum critical point is found where the ordering temperature of a phase such as ferromagnetism is pushed down to zero by adjusting a tuning parameter – such as pressure or doping. At this point there can be no energy barrier to forming short-lived pockets of (magnetic) order and these exotic fluctuations take over the system’s behaviour. It is common to find the emergence of new states of matter in this regime of the phase diagram.
The figure is from Coak et al. PNAS 117, 12707, where we used pressure to tune, for the first time, a quantum critical ferroelectric (rather than ferromagnet) - SrTiO3 - across its whole phase diagram, uncovering new exotic states of matter such as an instanton liquid phase. The next step now is to extend this same treatment to 2D ferroelectrics.
Magnetic structure
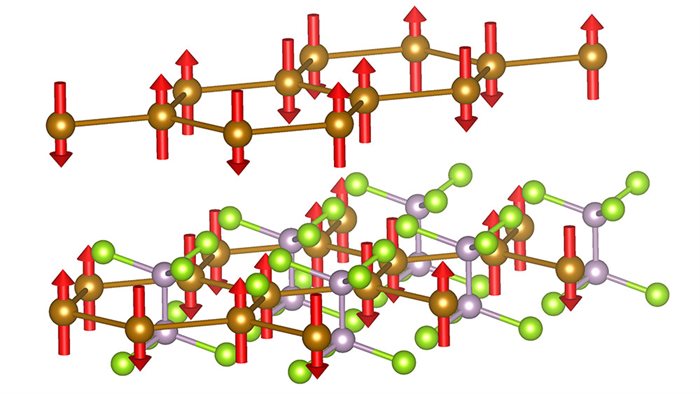
Magnetism is fundamentally different in the 2D limit. A 2D Ising-type (spins constrained along a single axis) magnet is able to form long-range order at finite temperatures, but more isotropic setups cannot - unless a tiny interplanar interaction is introduced to upset the balance. New types of magnetic arrangement can form, such as spin glasses and skyrmion lattices, and the behaviour of critical fluctuations as an ordering transition is approached can be strikingly different in low dimensions too. Traditionally it has been experimentally impossible to study magnetism in the real 2D regime, but new materials families and advancements in techniques are making it possible to study this physics for the first time.
Neutron scattering is the most powerful and direct tool in understanding the details of magnetism in materials. We have a long running and close relationship with the Institut Laue-Langevin in Grenoble to carry out these measurements and to keep pushing the boundaries of what kinds of experiment are technically achievable. One such example is our record-breaking high-pressure neutron diffraction on 2D antiferromagnet FePS3 up to 180 kbar of pressure (Coak et al. Phys. Rev. X 11, 011024) – the specialist equipment to carry out this challenging measurement is now routinely available to all users of the facility, unlocking swathes of new science.
Electrical transport
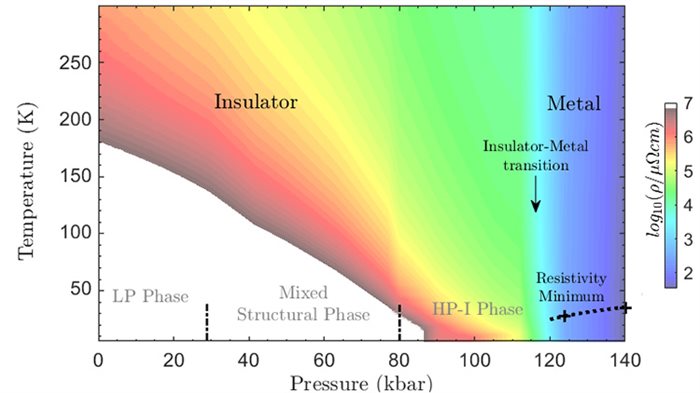
How the electrical resistivity, magnetoresistance and Hall effect of a crystal change with temperature, field and pressure give powerful insights into the microscopic detail of its interactions and its electronic structure. Many types of exotic order, or quantum critical fluctuations will modify the scattering lengths of electrons flying through the crystal lattice – so transport will pick up the changes as these states form.
A particular focus of our work is in fact on Insulator-Metal transitions in 2D materials, such as our discovery of the first Mott transition without a structural change in a 2D system, in VPS3 (Coak et al. npj Quantum Materials 4, 38), pictured. With no structural transition, this was driven solely by the slow increase of electron hopping between crystal planes as the system was tuned from 2D to 2.5D by hydrostatic pressure. Once metallised, VPS3 then has a low-temperature upturn in resistivity we are still working to understand.
Nanodevices and Heterostructures
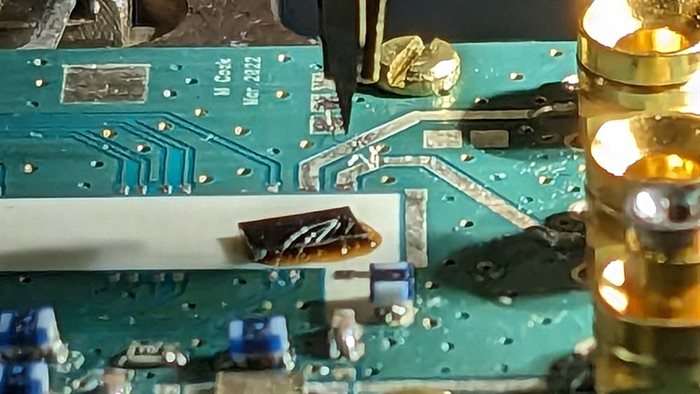
We are also exploring the fabrication and measurement of samples exfoliated down to be only a few atomic layers thick. How a bilayer or even a monolayer of a 2D magnetic material will be affected by applying pressure tuning is an unanswered question.
We can also stack up combinations of different 2D materials to form multifunctional heterostructures – for instance building an artificial multiferroic from separate magnetic and ferroelectric layers – and study their properties. We are working with electrical engineering groups and industry partners to explore exciting new technological applications for the new materials and phases we discover.