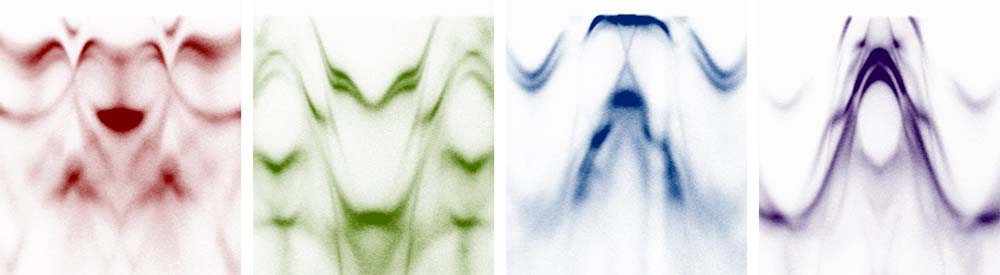
Our group specialises in the study of the electronic structure of quantum materials. We perform angle-resolved photoemission spectroscopy (ARPES) experiments which allows direct insight into the electronic structure, as well as the interactions affecting the electrons.
We are also affiliated with the Diamond Light Source, the UK national synchrotron, where we are developing the technical capabilities to apply uniaxial strain at the ARPES and other compatible beamlines.
PhD and postdoc opportunities
We perform experiments at large synchrotron facilities in the UK and abroad. These involve careful sample preparation and travel to synchrotrons for short periods of high-intensity data acquisition. You will gain experience with advanced methods of large data processing, which are of great use for both scientific and data science careers. We also welcome students wishing to develop engineering skills designing strain equipment for synchrotron experiments in collaboration with Diamond Light Source. For prospective research opportunities, contact Igor Marković to arrange a discussion. Open PhD studentships are listed on the central University Find a PhD page, but informal discussions are welcomed at any time.
Useful links
Dr Igor Marković's staff page
Dr Igor Marković’s research portal
contact: i.markovic@bham.ac.uk
Research
Angle-Resolved Photoemission Spectroscopy (ARPES)
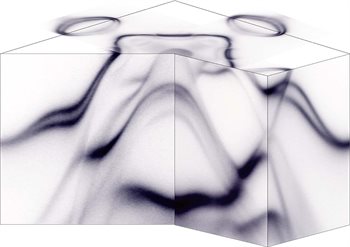
Angle-resolved photoemission spectroscopy (ARPES) provides insight into the momentum-dependent single-particle electronic structure and many-body interactions in crystalline solids. It relies on the photoelectric effect, whereby light is used to excite electrons out of the material, which are then detected such that the experiment records their emission angles and kinetic energies. Conservation of energy and momentum then allow us to use those parameters of the emitted photoelectrons to reconstruct the dispersion of the electronic states in the material. Beyond this basic approach, ARPES can also provide:
- orbital information, by utilising different polarisations of incident light
- spin-resolution, by employing spin-selective scattering processes in specialised detectors
- spatial variations of the electronic structure, provided a sufficiently small beam spot
- time-evolution of optical excitations, by using pump-probe setups to capture ultra-fast snapshots
We perform our experiments on user facilities in the UK and overseas. We are affiliated with the Diamond Light Source, but also use other synchrotron facilities, as well as the ARTEMIS experiment for time-resolved ARPES at the UK Central Laser Facility.
Interacting electron systems
Strong interactions of electrons with their surroundings, as well as one another, give rise to an entanglement of the charge, orbital, spin and lattice degrees of freedom in the electronic wavefunctions of the host material. In such cases, a multitude of degrees of freedom with similar energy scales provides a rich landscape of intertwined quantum orders and phenomena. Their interconnectedness then allows for small tuning perturbations to dramatically change the properties of the material and access novel exotic phases. Some examples include unconventional superconductivity, correlated electronic insulators, charge-density waves, quantum spin liquids, as well as multiferroics and non-collinear magnetic orders such as spin spirals and skyrmions.
Our research is primarily on the electronic phenomena arising from the interplay of spin-orbit coupling and crystalline symmetries. We are currently focussing on the nature of spin-orbit Mott transitions and the physics of the effective angular momentum manifolds in layered perovskite ruthenates and iridates, and lacunar spinels. Our goal is to better understand these phenomena and then explore how they are affected by tuning parameters such as chemical substitution and strain.
Topological electronic structure
The electronic states in crystals can sometimes have their energy levels inverted with respect to the atomic orbitals from which they are derived. Under certain circumstances, this leads to protected degeneracies of electronic bands, and the appearance of conductive electronic states at surfaces of bulk insulating materials. These are known as topological semimetals and topological insulators as these properties come from the nontrivial topology of their many-body electronic wavefunctions. Fundamentally, the electrons in topological bands represent a condensed matter realisation of exotic particles theoretically predicted in high-energy physics, such as Weyl and Majorana fermions, allowing for their investigation and application. Their transport properties show great potential for use in spintronic devices, as well as realising a potential materials platform for fault-tolerant quantum computing.
Spin- and angle-resolved photoemission spectroscopy is one of the primary techniques used in to identify and investigate topological materials, as it provides a direct view of the electronic band structure where the topological effects are most directly visible. Having discovered a 2D analogue of Weyl fermions in a nonsymmorphic square-net material, we are interested in how the symmetry of the crystal structure provides topological protection to electronic degeneracies, generating topological band structures.
Technique development
Controlled application of strain to quantum materials allows us to carefully tune the electronic hopping parameters, as well as alter their underlying symmetry. This provides a path to both investigate and drive novel exotic phases of matter and uniaxial strain experiments are gaining popularity across experimental condensed matter research.
We are partially affiliated with Diamond Light Source, the UK national synchrotron facility, where we are leading the development of a device for controllable application of uniaxial strain in ultra-high vacuum experimental environments to be used at the ARPES experimental beamline, but also at other compatible spectroscopy and scattering beamlines as well.