by Berta Bonet, Iseult Lynch and Stefan Krause
New research in our artificial stream mesocosms has shown that the increased temperatures predicted to occur by mid-century will lead to reduced aquatic biofilm diversity and enhanced susceptibility to other stressors. We found that combined exposure to elevated temperature and silver nanoparticles led to increased cell death in the biofilms of our artificial streams and a reduction of the surrounding protective matrix, as well as enhancing the toxicity of silver sulfide nanoparticles currently considered to be of low risk. As biofilms contribute to essential ecosystems services, such as water quality and nutrient recycling, monitoring of UK waters under the UK 25-year Environment plan and the River Basin Plans should include and prioritise biofilm health, and strategies to utilise biofilms for monitoring and remediation since there is a strong connection between healthy ecosystems and human health.
While for most of us, biofilms are the undesirable green/brown slime that cover buildings, boats, rocks and other surfaces in frequent contact with water, in their natural habitat, freshwater biofilms are an extremely hardworking part of the ecosystem, providing a range of essential services (often called ecosystems services) including water purification, carbon storage and nutrient cycling. Biofilms colonize any wet or submerged surface (substrate), and indeed recent advances in techniques for assessing microbial composition have shown that the biofilms lining pipes that bring drinking water into our homes may contribute to the high quality of drinking water, acting like the “good bacteria” in our guts by filtering out pollutants. Surface colonisation of biofilms is driven by extracellular polymeric substances (EPS) secreted by the bacteria, and this sticky mucus provides biofilms with stability and community structure, making them more resilient to pollution than individual microbes.
Biofilms are hugely variable in their composition, comprising algae, bacteria, and fungi, embedded in a protective mucus matrix (Figure 1), with each of the species having different functions and abilities. An established biofilm has a defined architecture, and biofilm-associated organisms differ from their planktonic (freely suspended) counterparts with respect to the genes that are expressed. Freshwater biofilms can also incorporate clay materials, suspended solids, and corrosion particles entrapped into their mucus matrix, and are well-known as sinks for pollutants (Bonet et al., 2012, 2014) thus aiding water purification. The different species within biofilms are closely associated and interact strongly with each other through secretion of signalling molecules (so-called quorum sensing). These interactions can be cooperative or competitive and are strongly affected by surrounding conditions; biofilms composition and structure change as they are exposed to extremes of flow, temperatures, light and nutrients in rivers and ponds.
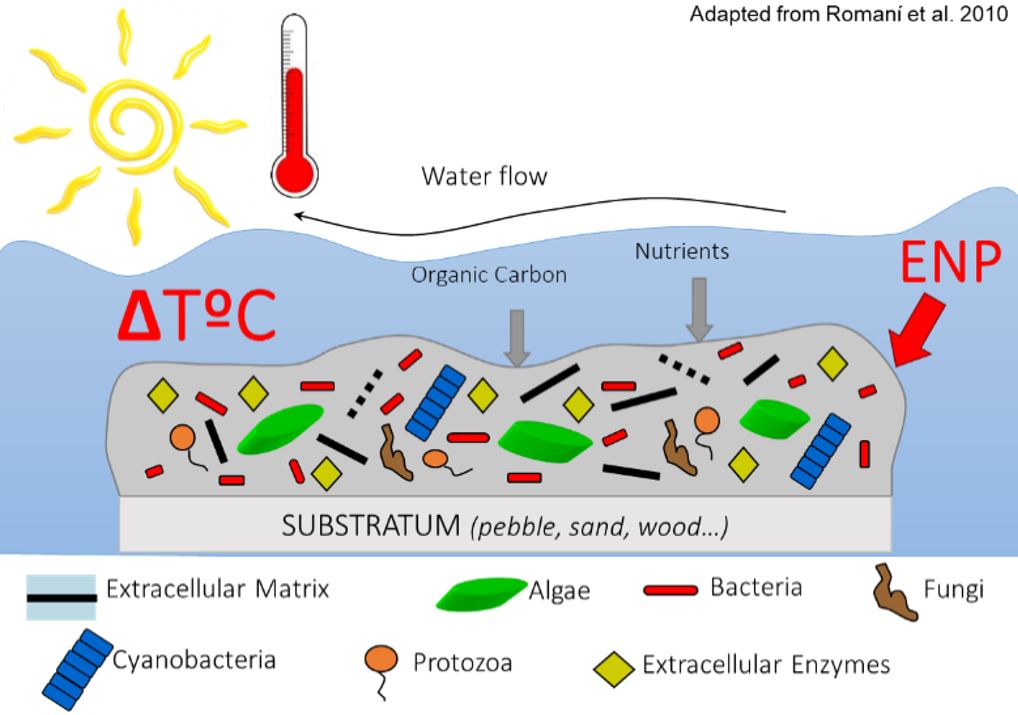
Figure 1: Schematic illustration of the impacts of increasing temperature (T) and interaction with engineered nanoparticles (ENP) in terms of their composition, structure, and function.
Aquatic ecosystems are threatened by multiple environmental stressors including pollutants and climate change. Climate models predict mean annual temperature to increase by 3.5ºC in air and 2.2–4.3ºC in stream waters by 2100, and increased frequency of extreme hydrological conditions including droughts and floods, with potentially devastating effects on freshwater ecosystems and their ecosystem services. These predictions are reinforced by the UK Climate Projections 2018 study, the most up to date assessment of how the UK may change over this century, which also suggests that under the highest emissions scenario, summer temperatures could be 5.4ºC hotter by 2070, with the chances of a summer as warm as 2018 (when daytime temperatures in parts of the country consistently topped 30ºC are around 50% by 2050. Even under a low emissions scenario, the Met Office data indicate that the UK will see an increase in the average yearly temperature of up to 2.3ºC by 2100. In freshwater systems, both temperature and hydrology are key controls on the rates of vital physiological processes such as respiration, growth, metabolism and enzymatic activities, and even small (e.g., 2ºC) shifts in temperature could markedly alter biofilm structure and functioning, while stream desiccation or drying would alter the nutrient cycle (as biofilms become more heterotrophic i.e., need to get nutrients by eating other organisms, and bacteria use more carbon and phosphorus for growth rather than nitrogen).
While considerable progress has been made in understanding the environmental impact of many stressors in recent years, new potentially powerful, toxicants such as engineered nanoparticles (ENPs) and microplastics arising from mis-managed plastic waste, continue to emerge whose effects on aquatic ecosystems remain poorly understood. The release of ENPs into the environment is accelerating due to their widespread applications in cosmetics, textiles, food packaging, building materials, electronics and more. Current regulatory testing utilises standardised over-simplified conditions, and does not factor in any enhanced organism susceptibility due to climate change or other stressors. The impact of combined climate change and particle impacts on individual species, for communities such as river biofilms, and for the essential ecosystems services they provide are critical questions that require answers. European environmental legislation such as the Water Framework Directive (WFD 2000/60/EC) recognises the need to manage the environmental threat posed by climate change coupled with contamination, but knowledge of new ENP and microplastics stressor effects is only beginning to emerge.
As a major step towards understanding the climate change-enhanced environmental impacts of ENPs in aquatic ecosystems, the Horizon 2020 funded project NanoTOX elucidated how much river warming affects fluvial biofilms structure and function, and how the presence of environmentally relevant concentrations of representative ENPs may further stress the communities (Figure 1). This was achieved through an innovative, interdisciplinary approach using an array of methods from the fields of ecotoxicology (ecology and toxicology), molecular biology, functional ecology and nanotechnology. Utilising UoB’s Environmental Change Outdoor Laboratory (or ECOLaboratory) and its recirculating streams at different temperatures, flows and pollution conditions, a large-scale mesocosms experiment was performed exposing biofilms to low concentration of ENPs at current and expected temperatures (18ºC vs. 25ºC). Since there is ongoing debate as to the role of nanoparticles versus dissolved ions, and the impacts of newly released (freshly dispersed) versus environmentally aged (transformed) nanoparticles, our experimental design included pristine silver nanoparticles (AgNP), silver ions as an ionic control (AgNO3) and a silver sulfide nanoparticle (Ag2S NP) designed to mimic the transformation that AgNPs undergo in the environment, such as during transport through wastewater treatment plants which are high in sulfide and a key route into the environment for nanoparticles.
The experiment involved 40 flumes of 60 Litres each, 20 set at 18 °C and 20 set at 24 °C, with 4 different pollutant treatments each: (i) untreated control, (ii) silver ENPs (AgNP, pristine ENP), (iii) silver sulphide ENP (Ag2SNP, aged ENP) and (iv) silver nitrate (AgNO3, ionic control Ag+), as shown in Figure 2. To assess differences over time, water and biofilm samples were collected just before adding the toxicants (0h) and after 1 and 3 days to assess acute effects, and after 14 and 30 days to assess chronic effects. Functional and structural analyses were performed according to the protocols developed within the project.
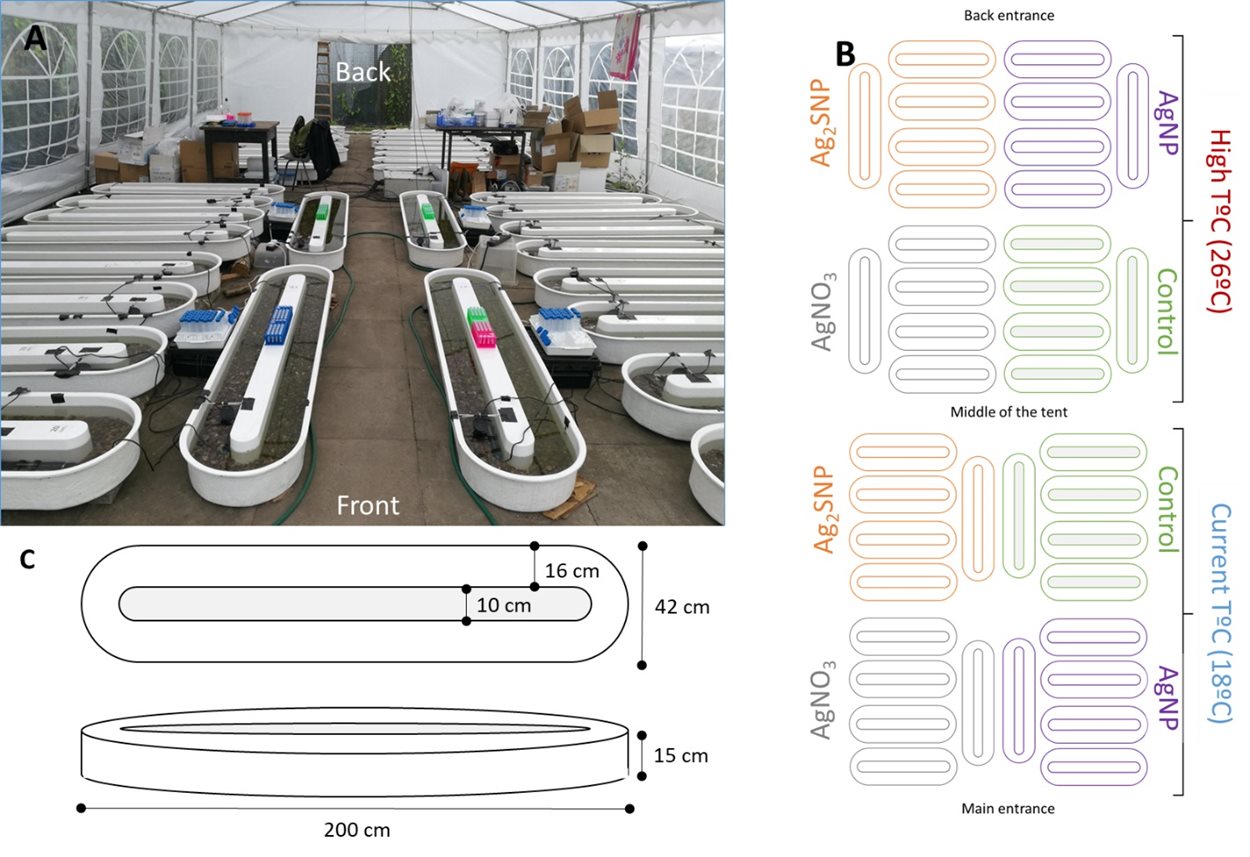
Figure 2. (A) a photo of a former ECOLaboratory and (B) a scheme of the mesocosms set-up used to perform the experiment. (C) Detail of flume dimensions.
Project results highlight that a temperature increase of 6 °C enhanced algal biomass, photosynthetic activity, and some enzyme activities while others decreased. Microscopic images showed a homogenisation of biofilm community composition (see Figure 3). These shifts should be taken seriously by river managers since they can modify the nutrient cycle in the freshwater systems as well as other ecosystem services, such their involvement in the global carbon budget, and thus have an important effect on the quality of water not only for the organisms who are living in the systems but also for human health. Both pristine and aged ENP agglomerated as soon as they were released into the system, due the content of divalent cations in water (calcium and magnesium). Even with such agglomeration, that could reduce the potential toxicity of AgENP, different toxic effects were observed between the different forms. AgNPs caused negative effects on fluvial biofilm respiration and on all diatom forms at 18 °C. Despite the effect on diatoms, AgNP biofilms retained or increased overall algal biomass and photosynthetic activity. Extracellular enzyme activity in biofilms (Phosphatase) was clearly affected over time by the Ag2S ENP and AgNO3 but not by Ag ENP. Phosphatase activity (related to phosphate degradation) was affected by aged ENP (Ag2SNP). These results are important since in theory these aged and unreactive ENP “should not cause” any biological effects and are considered to be of low toxicity. Increased temperature also affects ENPs properties such as solubility, dissolution and toxicity (Reidy et al, 2013), while flooding can (re-)mobilise the particles or enhance their sedimentation under drought conditions (as happens with natural particles). These results have important implications for the spreading of wastewater treatment plant sludge on agricultural soils, since aged ENP (Ag2S NP) in theory “should not cause” any biological effects, but under climate warming conditions had effects on fluvial biofilms and thus might also affect soil microbial communities and thus soil health and productivity.
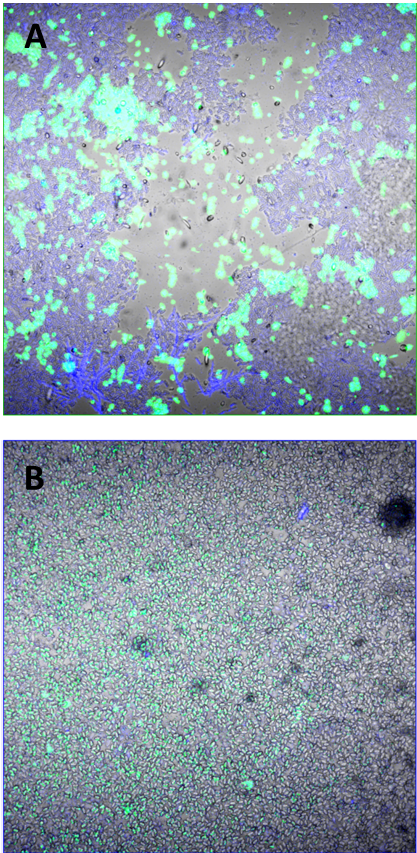
Figure 3. (A) Biofilm after 4 weeks at 18ºC and (B) at 25ºC, without any nanomaterials. Images show biofilm homogenisation at higher temperature and loss of extracellular polymeric substances.
Micro and nanoscale plastic particles have many features in common with ENP, including their particulate nature. The effect of temperature on microplastics’ physical properties (e.g., increasing density causing faster sedimentation) and surface properties (e.g., making them less hydrophobic), coupled with the aforementioned impacts of temperature on aquatic biofilms behaviour can affect the leaching rates of additives and plasticizers from microplastics. Thus, microplastics and their components will affect microbial communities and their ecosystem services, while the biofilms will alter the microplastics’ properties. There is thus a critical risk that global warming effects will markedly exacerbate the toxic effects of microplastics on fluvial biofilms, impacting on their function and provision of essential ecosystems services. Additionally, the role of biofilms in sequestering microplastics could be utilised as a low-cost strategy for their removal from water. Moreover, if specific plastics are found to age more effectively, or if biofilms are found to be effective barriers, this could be utilised more widely as a low-cost wastewater treatment strategy. Indeed, the first reports of utilising biofilms to trap microplastics for remediation, and of combining microbial biofilms and phytoremoediation via floating rafts, are beginning to appear in the literature, although again have not been assessed for their robustness under elevate climate conditions.
Our research is also exploring the impacts and opportunities presented by freshwater biofilms to act as indicators and mitigators of the ecosystems impacts of combined threats of particulate pollution and climate change. This knowledge will support policy makers involved in river restoration, waste management, environmental remediation and more, and will feed into the 25-year Environment plan. Indeed, it is striking that figures released by the Environment Agency showed that no UK river has achieved good chemical status, suggesting pollution from sewage discharge, chemicals and agriculture are having a huge impact on river quality, based on the more stringent test standards compared to those used in 2016 when 97% of rivers were judged to have good chemical status. Our data on the combined impacts of particulate pollution and climate change on fluvial biofilm structure and function indicate an urgent need to review freshwater monitoring guidelines - it is extremely important to not only monitor water physicochemistry to assess and manage freshwater systems (risk assessments), but also to include biological analyses of ecosystem functioning, which are crucial in order to understand if a system is polluted and how it is affected. Biofilm is a powerful bioindicator of ecosystem health and can detect the bioaccumulation of metals (and most likely also plastics or their additives) even when levels are under the detection limit in water. The forthcoming changes to the UK river monitoring network planned as part of the River Basin Management plans 2021, as well as the improving quality of using fluorescence-based water sensors enable the detection of metabolism of biofilms in-situ. Complementing these, our current research is focussing on developing in-situ analysis tool to detect microplastics in situ in river systems (e.g., our team are currently developing a low-cost field-deployable Raman system for microplastic quantification in rivers via the Institute for Global Innovation theme on “Water Challenges in a changing world”) offer exciting new opportunities for UK-wide monitoring of biofilm health and interactions with microplastics, to support a range of regulatory and policy visions including the circular economy and the efforts to improve river health.
This interdisciplinary project is a major first step in opening a new research line in climate-ecotoxicology, focussing specifically on biofilms as entry points to the food web and assessing ENP and microplastics impacts under current and future climate scenarios. Acting now, to monitor combined effects, and determine approaches to mitigate combined stressor effects on our critical river ecosystems is essential, and the solutions developed here are deployable in global south contexts also to support the achievement of Sustainable development goal 6 in providing clean water for all.